Fast Chargers
Planned roll-outs of fast chargers have highlighted the main issues and challenges for their design and development. Nick Flaherty reports.
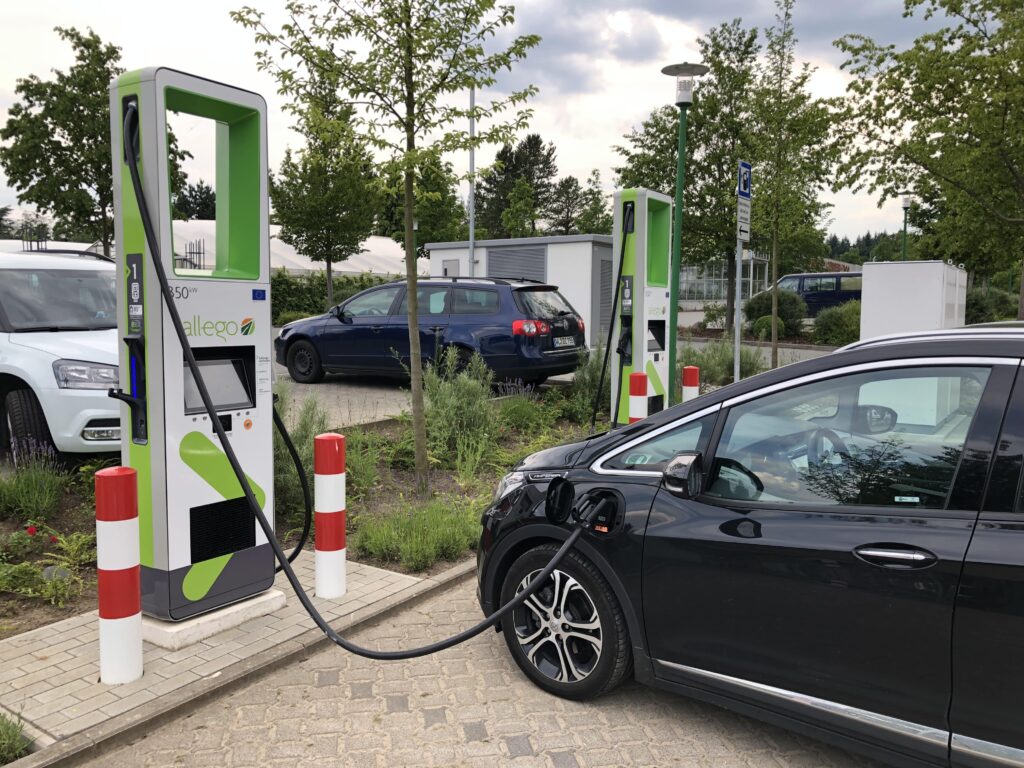
(Courtesy of Allego)
Power couples
Over the past five years, the number of fast chargers available worldwide has increased tenfold, and over the next five the aim is to have one fast charging station available for every 100 electric cars. These will provide 350- 450 kW to deliver a full charge in 10 to 15 minutes.
That has required an evolution of technologies in several areas to come together. New designs for the fastcharging electronics using materials such as silicon carbide (SiC) and gallium nitride (GaN) have improved the techniques for building transformers and cooling the chargers; high-current cables and connectors are also all enabling this roll-out.
As a result, the three primary concerns in DC fast-charger architectures are how to minimise the cooling requirement, deliver high power density and reduce overall system size. But there are continuing challenges, not least in the development of chargers with power levels of over 1 MW for commercial vehicles.
Typically, a high-power DC charger design converts an incoming threephase AC supply using an AC-DC stage and a number of DC-DC converter blocks to provide the DC voltage the vehicle being charged requires. A channel for data transfer is also included to provide information about the vehicle and the battery’s charging status, and there is growing demand for a secure data channel for measuring the energy used for billing purposes.
A key change from home chargers is the architecture of the fast charger. Rather than having a single AC-DC converter, designers are using converter blocks that can be produced in higher volumes and stacked together.
“Most charger designs are going this way,” says Stephan Hell, CTO of r&d at charger supplier Allego. “It means that if one unit fails the charger can still produce power, even if it’s not full power.”
This reliability is even more important in high-power chargers on motorways, also called the corridor application, where it is essential to have working chargers all the time as the next ones could be 80 km away. In a city, if the charger is not working then there’ll be another one within 2 km.
The power conversion rate is another important specification. Hell says, “All suppliers are on the same efficiency level, but we have to consider 97% efficiency versus 98%.”
It is then up to every charge point operator to decide whether to support 350 kW outlets, as there are currently no commercial cars that use this level. The maximum at the moment is 260 kW, in the Porsche Taycan, but charger suppliers are planning for future systems that will require that 350 kW level of charging.
Power from the grid might not be available at that level, so operators are looking to install six 150 kW chargers rather than six 350 kW, but provide the ability to combine the chargers. That would then allow one outlet to provide more than 150 kW while others are limited to 100 kW.
For heavy trucks and electric buses the charging is still an issue. Hell says, “There is an initial proposal for new connectors to reach megawatt charging, but that is still ongoing. This could be a breakthrough to equip heavy trucks with electric power.”
The European standard for highpower connectors, CCS, will be suitable for the next 10-20 years, says Hell. “Then you are talking about 200 kW of capacity, and then the industry will have to think about doubling the charger power to maintain the charging time.”
There are still issues with testing and compatibility though, so that every car works with every charger. “There are some details where we detect issues, but this is a learning curve that we can use for the next generation of cars coming up,” Hell says.
Charger architectures
The architecture of the fast charger has evolved into two elements – an active front-end AC-DC converter that can use different topologies, including power factor correction (PFC), and a number of smaller DC-DC power blocks.
“We see the complexity of the charging ecosystem as increasing significantly,” says Stefano Gallinaro, general manager of the energy business unit at Analog Devices (ADI).
The AC-DC stage can use a fullbridge topology with six silicon IGBT devices, although a newer topology called a Vienna rectifier has become a popular option for the front-end AC-DC converter. The Vienna can use the latest high-frequency SiC switches.
“We don’t see the need for advanced topologies at the moment,” Gallinaro says. “In the AC-DC converter we see SiC Vienna rectifier topologies having the best trade-off, or a three-level, sixIGBT topology.
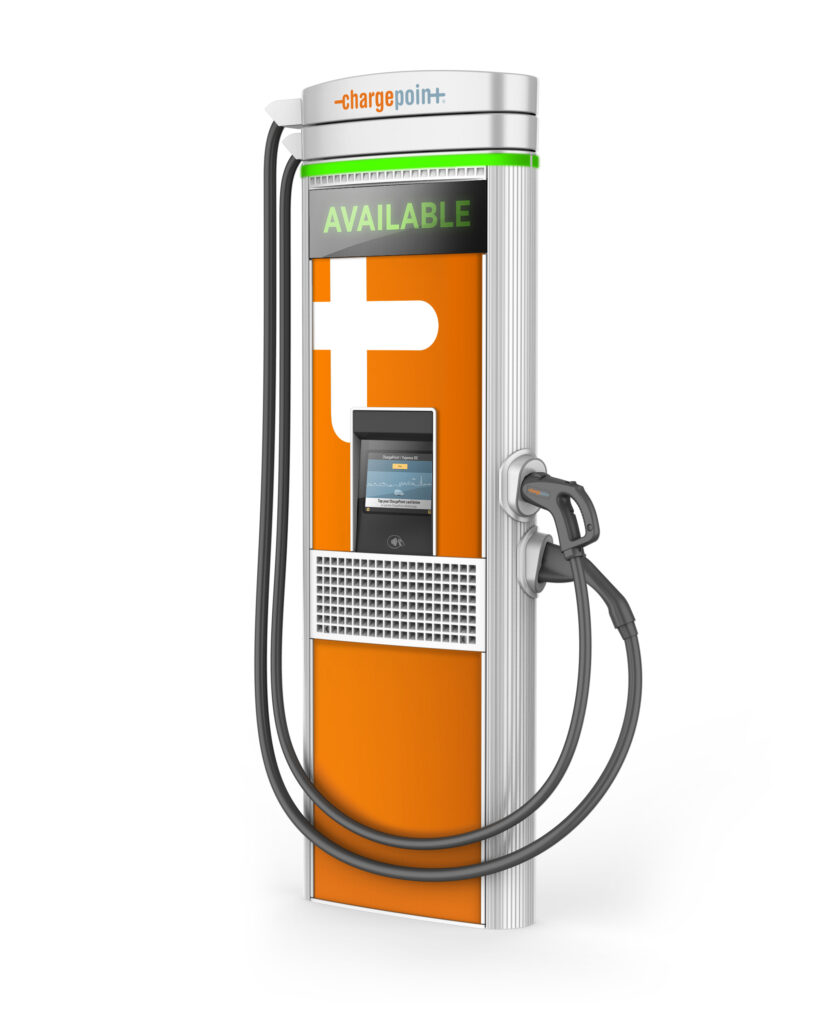
(Courtesy of Chargepoint)
“In some more complex solutions, with the energy storage system close to the charging station, that PFC first stage might act as a booster to avoid the two-stage conversion. The DC-DC block is then working with a limited voltage range, but there we see the full bridge as still effective, although it is an expensive solution.”
In the PFC stage a six-IGBT fullbridge or Vienna rectifier has a hard commutation of the switch, so the event switch loses switching energy. What limits the switching frequency is the losses. There are some other limits related to the EMI levels as there is a forbidden range from 150 kHz, meaning no hard switching above that frequency, and even above 50 kHz there can be issues with third-order harmonics.
“These losses are the strongest limitation in the switching frequency in the PFC,” says Vladimir Scarpa, vertical marketing engineer for power applications at STMicroelectronics. “In the DC-DC stage I can use a soft switch resonant converter. The advantage is that I can bring the current and voltage to zero when switching so the losses and EMI are much lower. The design can therefore go well above 100 kHz – designs running up to 400 kHz are already on the market.”
Efficiency is an important point for these designs. “I would not accept a system below 90% efficiency, as that’s 10% losses. For a petrol-engined car that’s like throwing out 5 litres from a full tank, and no-one will accept that,” Scarpa says.
“There is a push for efficiency up to a certain point – systems are at 98% for the Vienna and the DC-DC converter. With the two-stage architecture that gives a 96% total efficiency. For the P6 bridge it’s more or less the same.”
The SiC switches help to improve the efficiency. “We work with the SiC makers to provide gate drivers that bring the highest three-phase interleaved DC-DC converter running at 70-80 kHz per phase to give a total of 240 kHz,” Gallinaro says. That gives the best trade-off in cost and performance. SiC devices can go up to 150 kHz, but above that the design challenges are hard, so the sweet spot is 70-120 kHz.
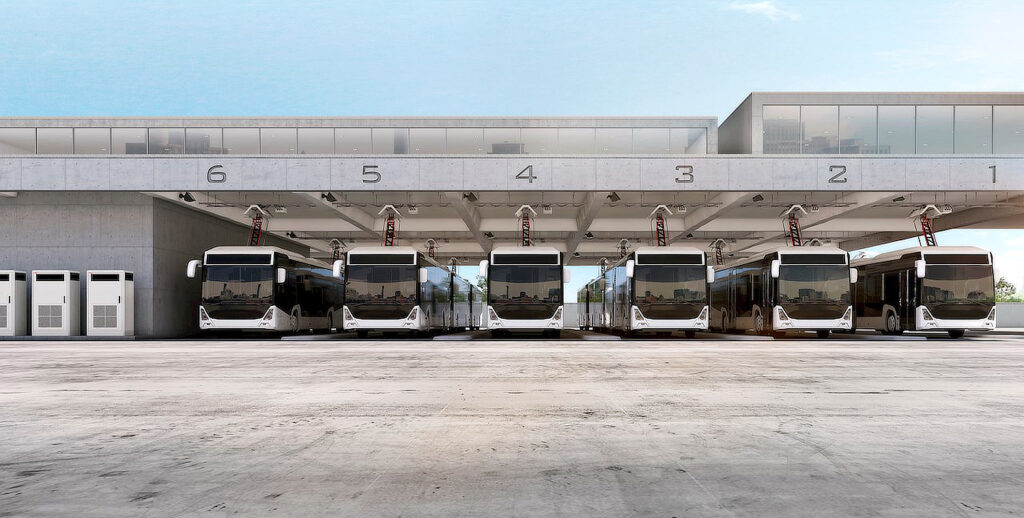
(Courtesy of ABB)
“With GaN the switching speed can be much higher – we have customers at 8 kHz but also from 150 kHz to 1 MHz,” Gallinaro says.
The coils and capacitors are not yet available for systems over 1 MHz, he says. “We expect the frequency will go up year on year as new coils, inductors and capacitors come to market.”
The challenge is to increase the lifetime of the systems, he says. While it is possible to design a very expensive, high-performance SiC charger with a high-capacity storage unit – at twice the cost of an IGBT charger – there is the question of how customers recover the cost. Increased efficiency is one way, but so is a 30% longer lifetime. That comes from having accurate measurements and monitoring.
GaN devices are still early in their technology development compared to SiC. “GaN on silicon is a help as it’s more common and easier to handle, but there will still need to be several years of evaluation and testing for GaN before the big customers jump in,” Gallinaro says.
Having the GaN switches built in a silicon substrate means the base technology is similar to SiC, but the key difference is how the system is cooled and managed.
“Once the die is packaged you get a high power density switch that is hard to cool, so it’s very easy to accidentally create hotspots with the smaller GaN die,” Gallinaro says. “Then you have a high power density charger with hotspots that are not immediately apparent but show up after maybe five years of operation.”
Chip makers are also now producing these DC-DC power blocks. Gallinaro says, “We were thinking about making complete subsystems. We work with start-ups’ r&d on building blocks up to 30 kW and use them for the big system integrators or as reference designs for selected customers.”
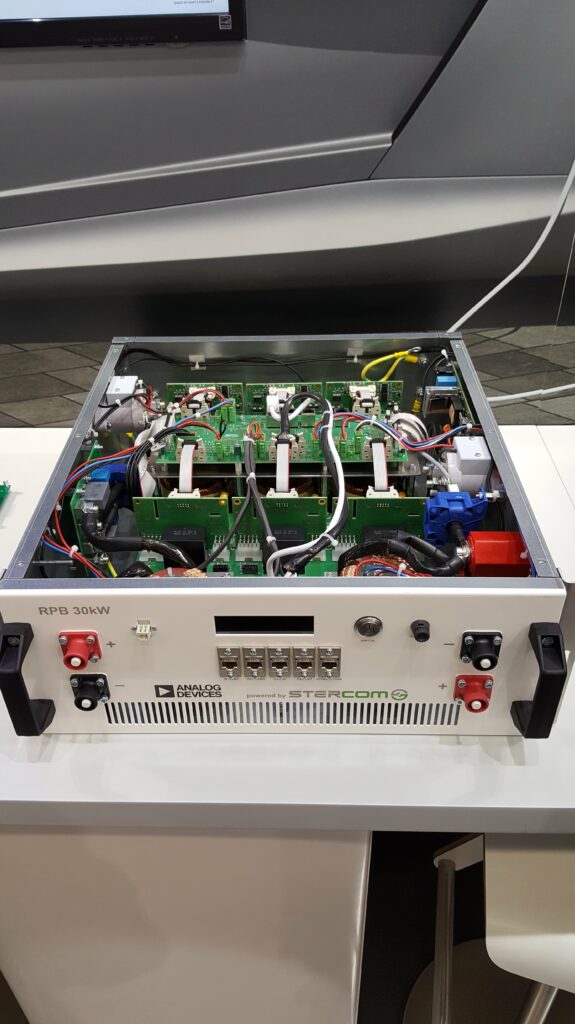
(Courtesy of Analog Devices)
STMicroelectronics is also developing its own power blocks as products, ranging from 20 to 50 kW, using its integrated modules with SiC in different configurations such as half- or fullbridge. “All charger makers are going in that direction, not the big 150 kW single system,” Scarpa says.
The most popular approach to using the blocks is in a column or rack. The blocks share the input voltage and parallel the output voltage. Having more blocks increases the overall efficiency of the system, as every one of them has an efficiency curve with a typical peak efficiency of 40-60%.
“That means it’s more interesting to use all the blocks and reduce the power each block is delivering, rather than use each one at full power,” Scarpa says. “It also means you are not overstressing individual blocks.”
This also supports higher voltages. “There isn’t a single battery pack level in terms of voltage – we talk about 400 V but that is increasing to 800 V, and the same charger has to support both, so you can use two power blocks to cover these two output levels,” he says.
Transformers
The transformer used in the PFC stage is also changing.
As an example, Andrea Polti, product manager for transformers at Murata, says, “We have developed a new winding technique that we are patenting. The winding involves using flat wire rather than tape windings or lit wire with multiple strands. With a flat wire we are bending on a different axis, on the narrow edge, so you get a helical winding like a spring.”
The advantage of this approach comes at higher frequencies. The higher the frequency, the more redundant the core of the conductor becomes, as the field is all in the surface. That is why litz wire is used, but it is expensive and cylindrical. Flat wire uses the winding window more effectively, and the straight edges provide more effective cooling with heat sinks or liquids.
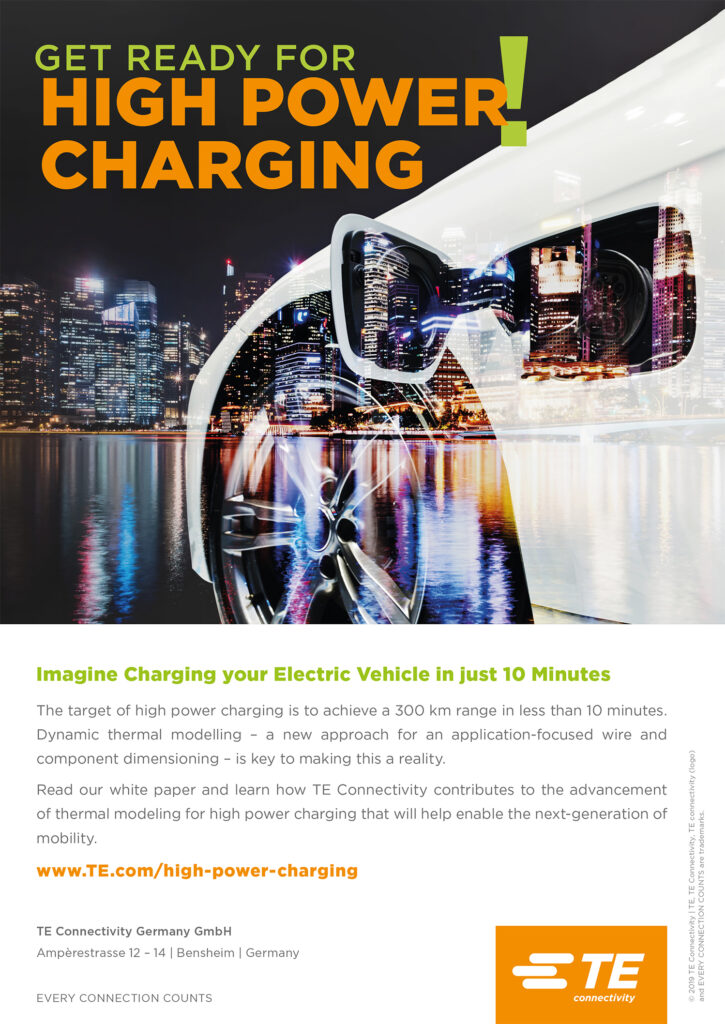
“With the windings, the key is in the way we form them,” Polti says. “We are putting the primary and secondary side by side all the way through. That negates the proximity effect that comes from eddy currents, and because of that, this technology is scalable from a few hundred kilowatts to megawatts.
“The challenge now though is to build it. We form the wire and put the two windings together. Because they are preformed you can twist them into each other, like intertwined springs.”
Another challenge is that the magnetic field builds up in the primary, which can lower the transformer’s performance, but the intertwined coils prevent this. As this winding supports higher frequencies, it allows designers to use the benefits of SiC.
Murata did a comparison test using three units at 100 kW: one with the new winding, one with tape winding and one with lit wire. In the tape-wound design, thermal runway occurred at a much lower power rating, while with the lit wire it reached 70 kW before the core became saturated. The tests showed Murata could use the technology to replace parallel multiple transformers in fast chargers starting at 150 kW and then 350 kW.
Connectors
Cooling is one of the major challenges for the high-speed charging cables and connectors. “For a 500 A, 1000 V system at +50 C, the challenge is in making the cable connector light and easy to handle for the user,” explains Max Goldi, market manager industry in the Low Frequency Division at Huber+Suhner.
That means reducing the copper cross-section to keep the temperature down, and using a synthetic dielectric coolant through the cable and connector.
The coolant flows through a pipe down the cable into the connector, cools the electrical contacts in the connector first and on the way back cools the copper cables carrying the power. “We are using a dielectric synthetic coolant, so there are no insulation issues and no risks if something goes wrong. As we are cooling directly on the copper, the efficiency is high,” Goldi says.
The coolant is pumped from a tank in the charger column at a pressure of 5 bar, using a heat exchanger to remove the heat as the coolant returns in what is a closed-loop system. The size of the tank depends on the length of the charging cable. The cable also has two or four signal lines alongside the power lines and the pipe that carries the coolant.
The standard design for CCS 1 and CCS 2 connectors in Europe is limited to 500 A. “In the lab we can run 600 or 700 A if the environment is not too hot, but it’s the design of connector that limits the power,” Goldi says. “That is down to the pin creepage distance.”
A new version of the Chademo connector standard used in Japan and China is being developed to carry 600- 800 A. That would use bigger contact pins in the connector with the same coolant, a higher flow rate and better heat transfer in the column.
Another issue is the length of the cable, as the longer the cable the more heat is generated. The maximum length for commercial chargers is 8 m, with 10 m in the lab, although the cable should be as short as possible. All the new fast-charger designs use a retraction system or ensure that the cables don’t touch the ground.
A new generation of connector is coming next year, which will reduce the weight from 1.4 kg to 1 kg and have integrated metering.
“One of the new things is preparing for metering systems,” Goldi says. “Operators want to measure the power at the pins in the connector. We can measure the voltage drop on the connectors and bring that back to the charger, then connect to the metering system.”
This needs two additional data lines in the cable, as the data cannot be mixed with the temperature data and comms with the vehicle.
There is also the issue of supporting both CCS and Chademo-style connectors.
“For passenger electric vehicles we have a maximum power of 450 kW, which can be carried by the CCS connector, and that’s more than enough for a 75-100 kWh battery pack,” Hell says. “With a 450 kW outlet, that cuts the charging time to 15-20 minutes, which is more than comfortable for this type of driving.
“At Allego we will support any EV driver, so we have to find an economic way to integrate Chademo as well. For a high-power charger, one of the set-ups we have designed is to have a kiosk with a cooled CCS cable with 350 kW on one side and a non-cooled Chademo cable up to 100 kW on the other. That means you can use both connectors at the same time – there are a lot of Nissan and Mitsubishi drivers out there using Chademo connectors.
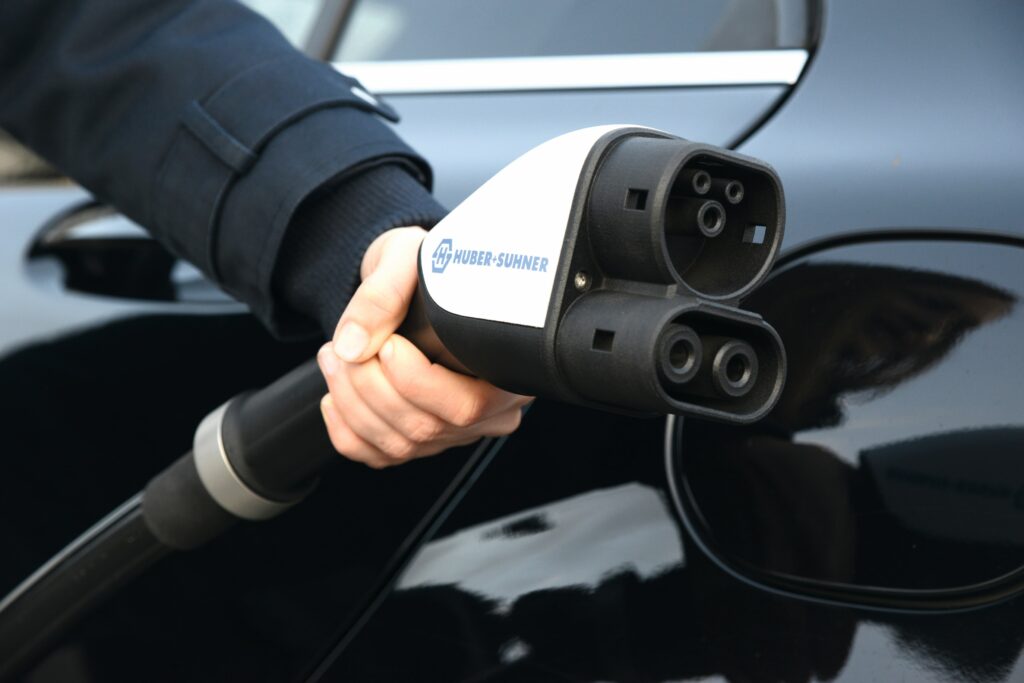
(Courtesy of Huber+Suhner)
Metering
More systems are requiring redundant metering to be available all the time, Gallinaro says. “Customers have a certified AC meter connected to the grid, but they want the metering values from the DC-DC converter block, as well as the metering near the connector, to analyse the flux of energy between the grid, PFC, DC-DC and the bus.
“For us this is a new challenge. We see the need for a DC metering system which is accurate and fast, and which can be used to prevent arcing and issues linked to the DC current.”
“We see the market as being pretty fragmented on metering at the moment, and we hope regulation will drive the market to a definition of a certified unit. Each charger company is looking to create its own systems to gather as much information as possible, but they don’t always know what is needed for analysis for consistent results. Some r&d institutes are simulating which data is really needed for good information out of the system.”
Grid
The grid connection to the fast chargers is also important.
Installers are looking at adding a battery-based energy storage (ESS) module to boost the power. “When you have multiple DC chargers on a street, you will have the issue of draining too much power from the grid,” Gallinaro says.
“For example, if you have perhaps five 800 V, 200 kW vehicles charging, you will need more than 1 MW from the grid, with a peak of 2 MW. The solution there is not about the most efficient components but an interconnection between the charging system and the grid, and a buffer that can limit that peak requirement by using an ESS This can be new batteries or a second life for used electric car battery packs.”
That requires more complex management – knowing when to charge the cars at the maximum rate, when to use the ESS at its maximum, and when to use the grid, solar or wind energy to make sure the grid is balanced.
The ESS is also the most expensive part of the system. The cost of a 2 MWh battery ESS will be much higher than the power conversion, so maximising its lifetime is essential.
The ESS also has to communicate with the DC chargers and the cloud management software, transferring all the data back and forth. That means the complexity of the system is moving up the stack, including the algorithms to monitor the state of charge and state of health of the ESS.
ONLINE PARTNERS
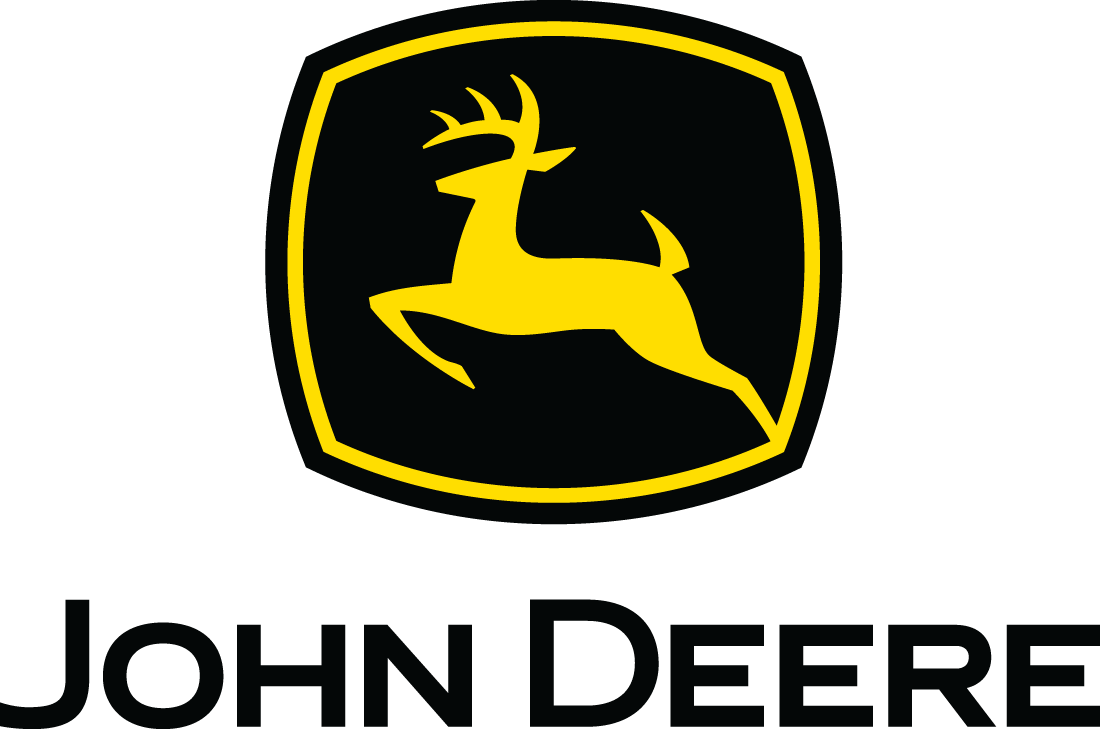
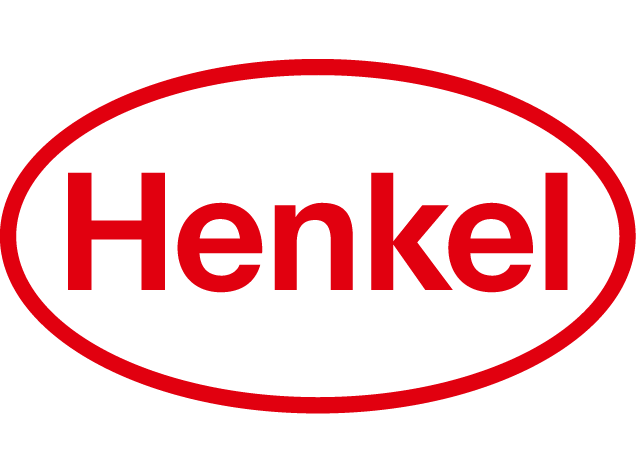
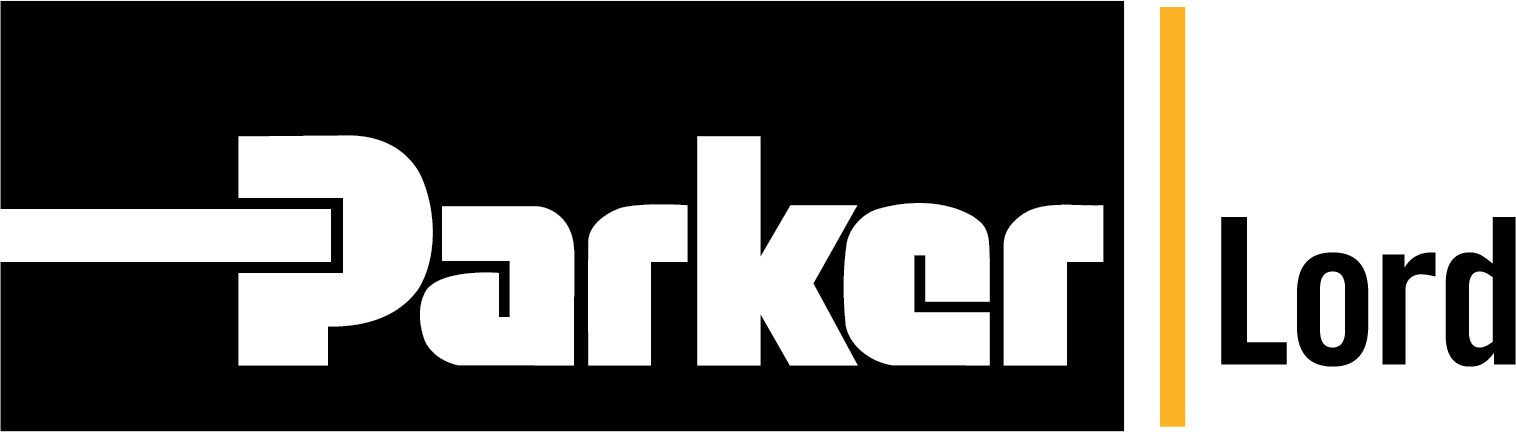
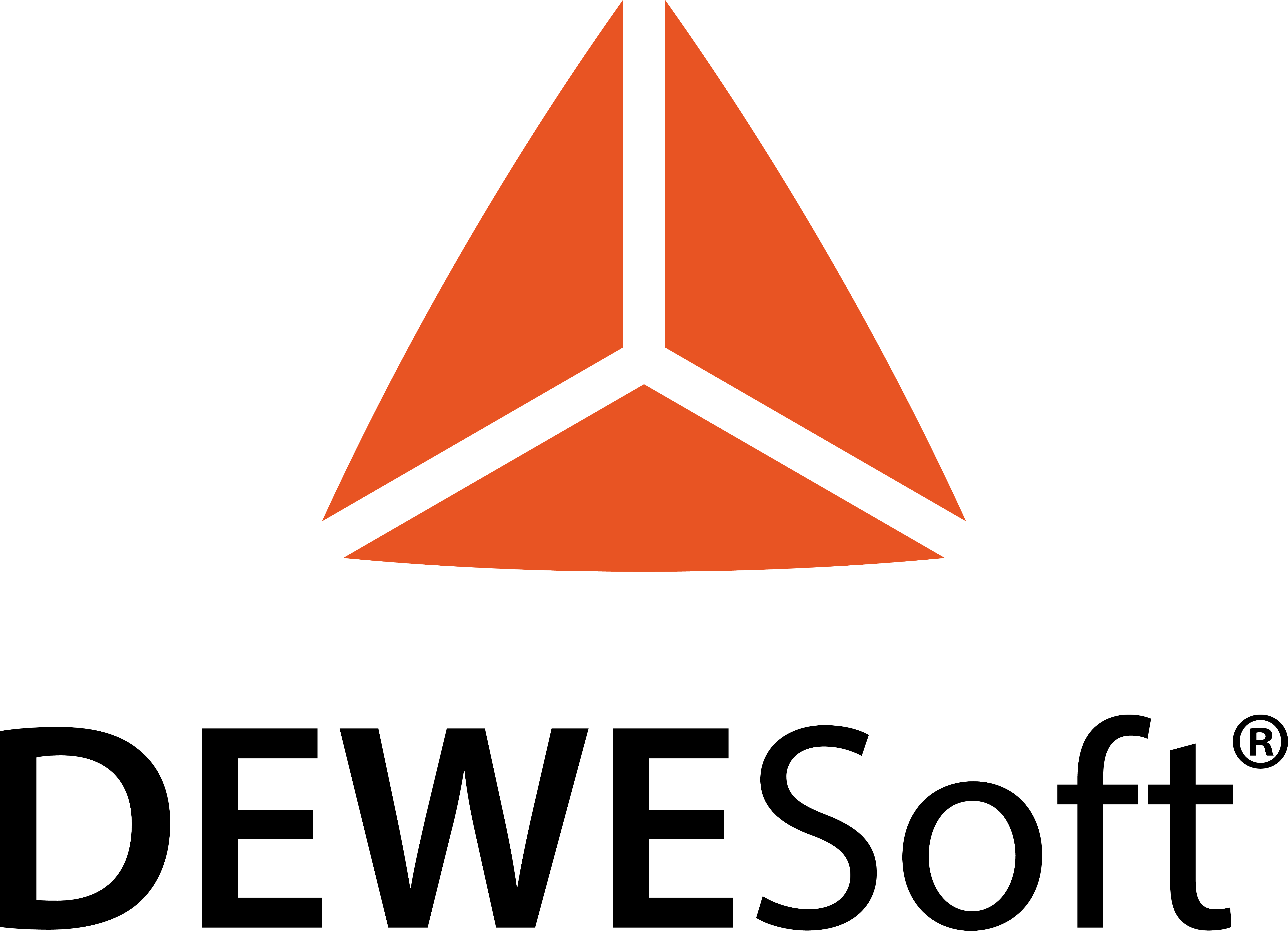
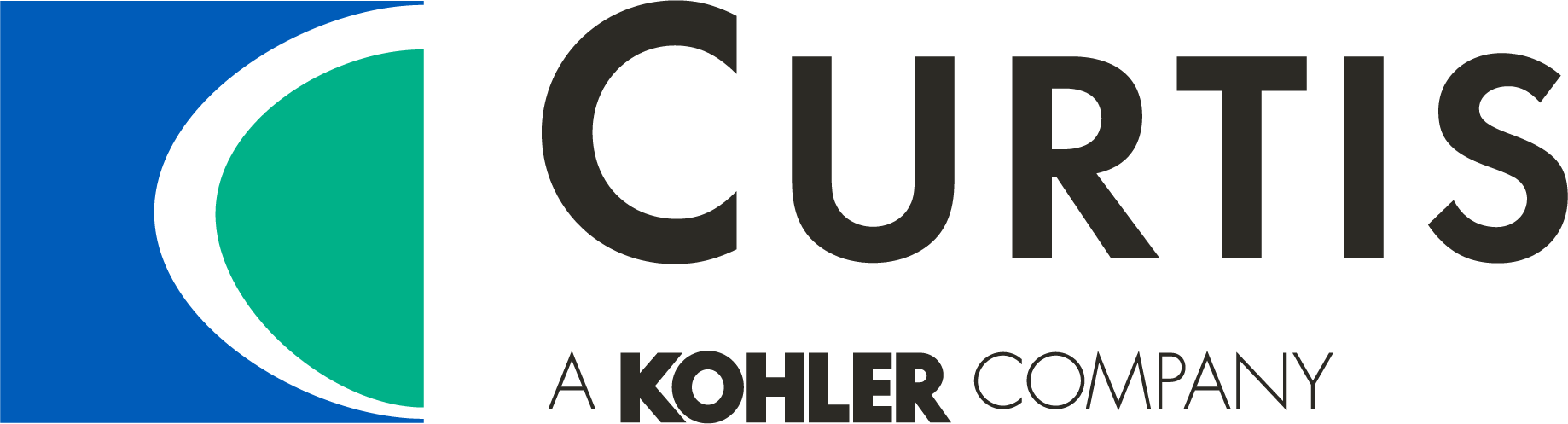
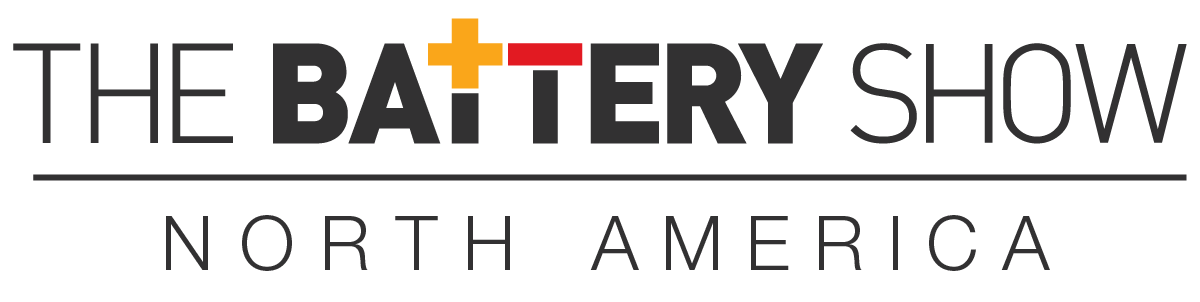