Wide bandgap semiconductors: comparing SiC and GaN for electric vehicles
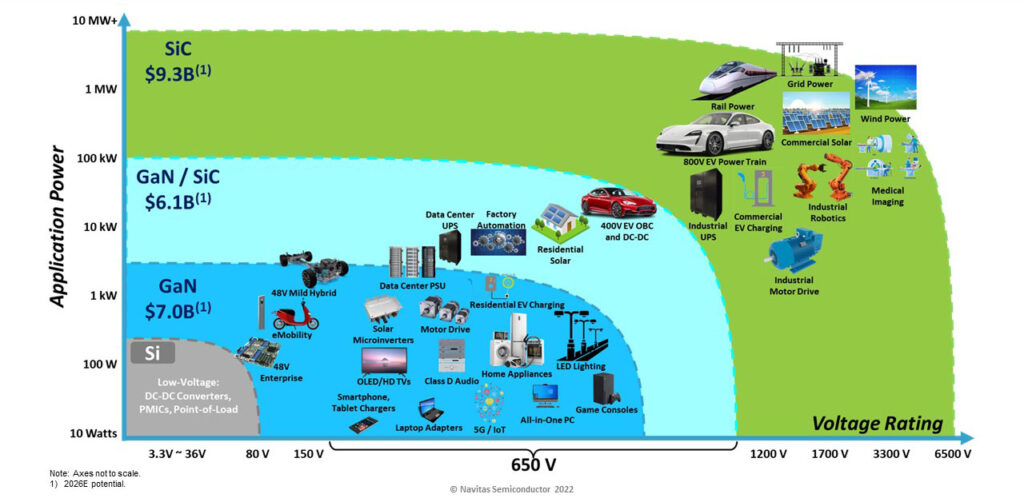
As system voltages in EV powertrains increase to enable the use of higher power levels without creating excessive currents and the associated energy losses and heat dissipation problems, so does the importance of wide bandgap semiconductors, writes Peter Donaldson. Ones such as silicon carbide (SiC) and gallium nitride (GaN) in applications such as traction inverters, DC-DC converters and onboard chargers.
The big question, then, is which would win in a fight? The answer depends heavily on the venue.
In crystalline solids – particularly semiconductors – electrons are arranged in bands representing different energy levels, two of which are essential to electronics: the valence band and the conduction band. The valence band is the highest energy band that is fully occupied by electrons at absolute zero, as they are tightly bound to the atoms in the crystal lattice. Immediately above the valence band is the conduction band, where electrons are free to move and so can participate in electrical conduction.
For insulators and semiconductors, the conduction band is empty at absolute zero. Expressed in electron-volts (eV), the difference in energy between the valence band and the conduction band is known as the bandgap, and it determines the material’s ability to act as an insulator, a semiconductor, or a conductor. A wide bandgap semiconductor can be thought of as a material with a bandgap wider than that of silicon.
Having a wide bandgap means that it takes more energy to move an electron from the valence band into the conduction band, a property desirable for many reasons. A wide bandgap usually goes along with a higher breakdown voltage, which is the voltage at which a device experiences a transition from a non-conductive state to a conductive state, allowing for higher operating voltages. A wide bandgap is also often associated with high electron mobility, which translates into higher switching speeds and lower switching losses, improving overall performance and efficiency.
A wide bandgap also tends to bring higher thermal conductivity (SiC is particularly good here), which helps to dissipate heat more efficiently, easing thermal management, along with tolerance of higher operating temperatures without loss of performance and an ability to operate efficiently over a wide temperature range. The combination of higher breakdown voltage, faster switching, and lower losses contributes to better overall efficiency in power conversion applications.
Inevitably, these advantages come with some drawbacks, including more costly and expensive manufacturing, relative technological immaturity, and less well-established supply chains. Further, integrating devices based on wide bandgap materials into existing power electronic systems might require changes to the overall architecture and to control algorithms, and could cause problems of compatibility with other components.
Back to the fight metaphor, the two semiconductors weigh in with very similar bandgap numbers, with SiC ranging from 2.36 to 3.23 eV and GaN with a slight advantage at 3.4 eV.
If the venue is a high-voltage, high current system such as an automotive or locomotive traction inverter, for example, SiC gets to dance around the ring holding the belt thanks to its much higher breakdown voltage of between 2 and 10 megavolts per centimetre (MV/cm) compared with GaN’s 1 to 3 MV/cm. SiC MOSFETs are available at ratings up to 2000V and with high current capabilities thanks to the material’s higher electrical and thermal conductivity. Today’s GaN devices are limited to about 650V.
If, however, the venue is a system such as a fast onboard charger, a DC-DC converter, a power distribution module or a wireless charging system, then the higher electron mobility and consequent faster switching of GaN devices can result in more energy efficient, lighter and more compact solutions.
As a postscript, neither SiC nor GaN is the semiconducting heavyweight champion when it comes to bandgap, as both are beaten by gallium oxide at 4.6 to 4.9 eV, diamond at 5.5 eV, boron nitride at 5.9 eV and aluminium nitride at 6.2 eV. But, as always, there’s more to the story than that.
Click here to read the latest issue of E-Mobility Engineering.
ONLINE PARTNERS
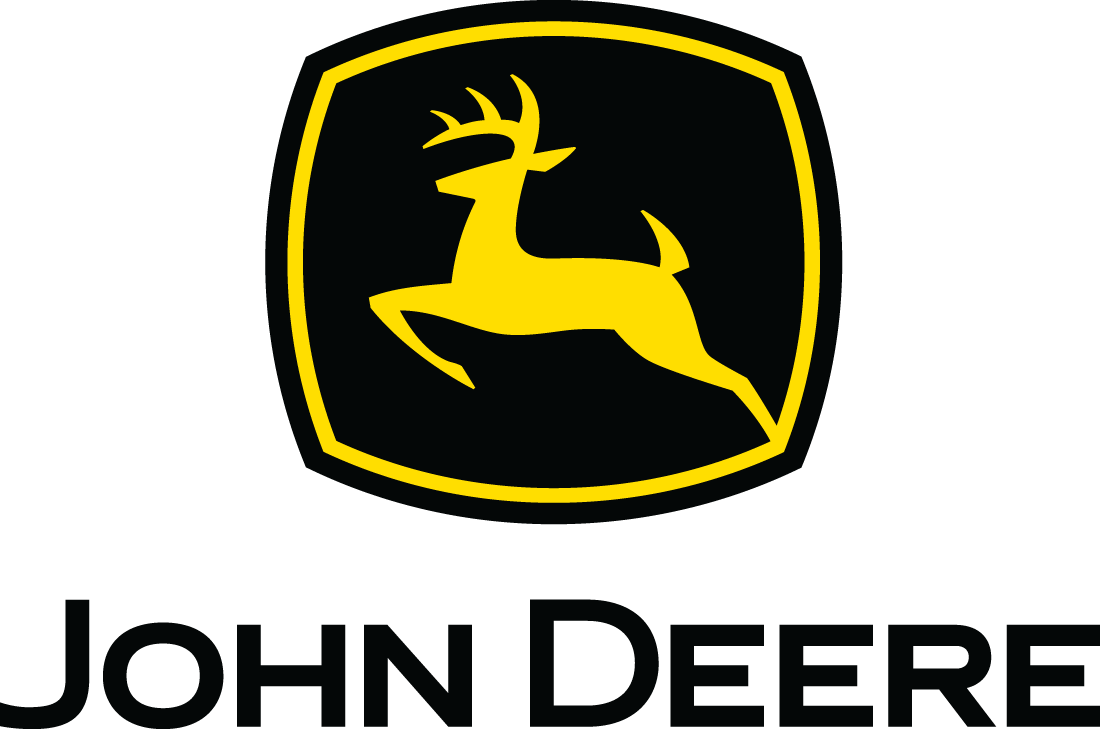
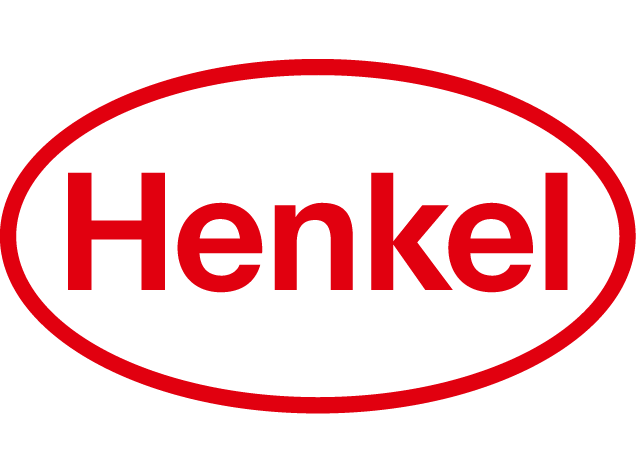
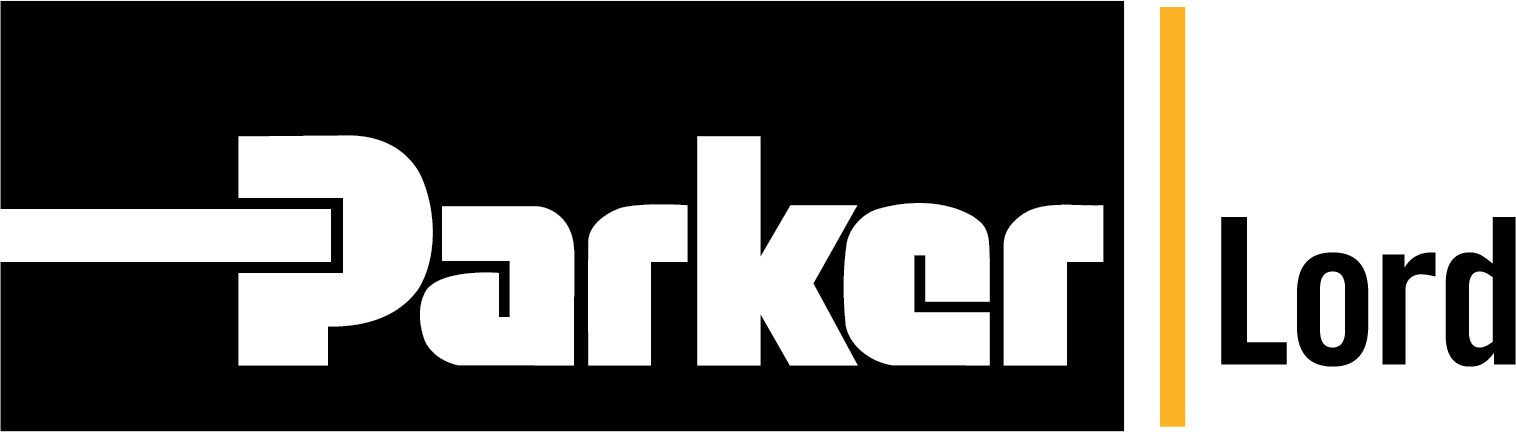
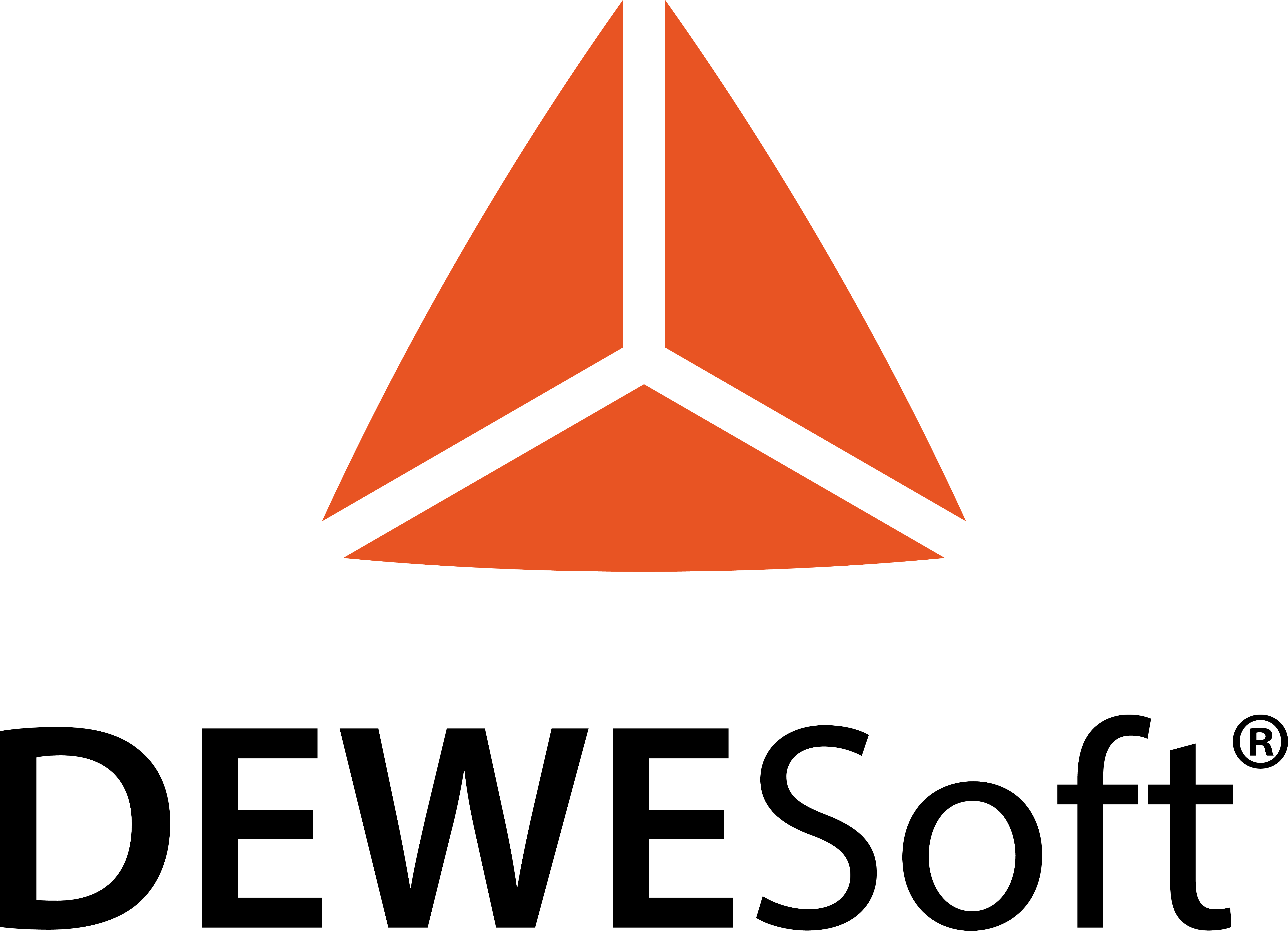
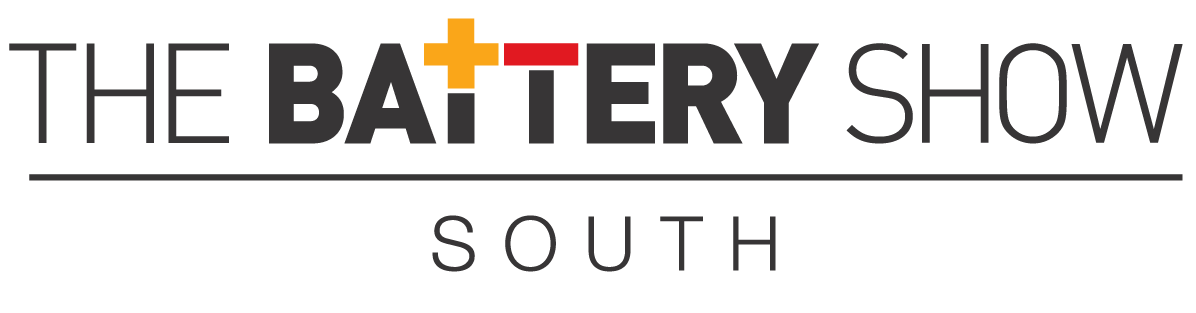
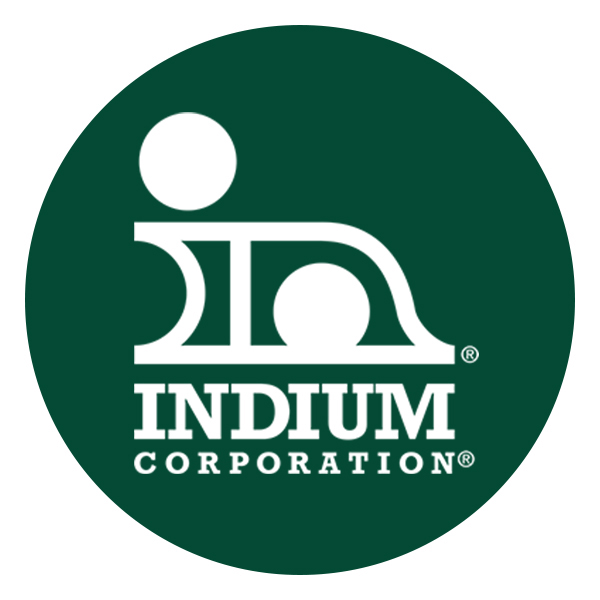
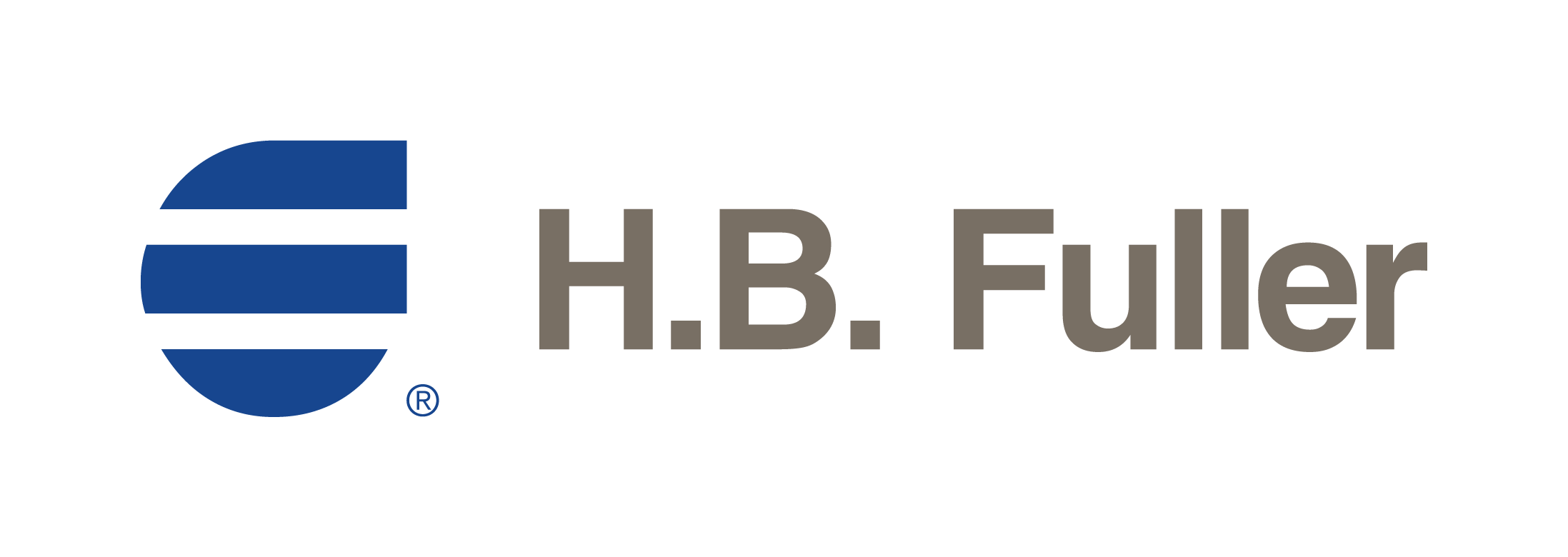